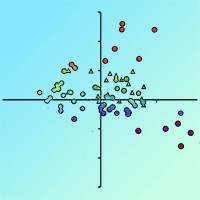
The effect of soil conditions on submountain site suitability for Norway spruce (Picea abies Karst.) in Central Europe
iForest - Biogeosciences and Forestry, Volume 16, Issue 4, Pages 210-217 (2023)
doi: https://doi.org/10.3832/ifor4262-016
Published: Jul 31, 2023 - Copyright © 2023 SISEF
Research Articles
Abstract
Norway spruce (Picea abies [L.] Karst.) occurred rarely at submountain altitudes before reaching its present position as the most important economic tree species in Central Europe. Spruce cultivation outside of natural sites is under constant threat from harmful agents. In this study, we focused on the indication of potentially suitable planting sites for Norway spruce in areas of protected submountain (< 700 m a.s.l) populations using linear discriminant analysis (LDA) of soil properties in oak-beech, mixed and spruce stands in the territory of the Czech Republic. The soil A-horizon properties in mixed stands were more similar to those in spruce than in broadleaved stands; stand mixtures resulted in lower soil property separability than the localization of individual populations (57% and 85%, respectively). The suitable sites were defined by acid phosphomonoesterase > 151 µg hour-1, soil porosity > 63%, aeration > 53%, bulk density < 0.86 g cm-3, cation exchange capacity < 18 cmol+ kg-1, Corg< 9% and Ntot< 0.5% in clusters comprising the Bohemian highlands, North Bohemian rock cities and the Outer Western Carpathians. The LDA of soil properties offered a useful tool for assessing both naturalness and forest threats to support sustainable management.
Keywords
Site Series, Spruce Cultivation, Soil Organic Matter, Extracellular Enzyme Activity
Introduction
Norway spruce (Picea abies [L.] Karst.) is one of the most important European coniferous tree species from an economic perspective ([25]). While this species has economic importance within areas of natural occurrence, not all sites are entirely suitable for long-term survival. Spruce cultivation outside of its natural sites is constantly under threat from harmful agents ([27]). The establishment of economically suitable spruce sites relies on a combination of sustainable forestry processes that increase yield sustainability and minimum pest control measures ([35]). However, environmental change affects suitable conditions for all living organisms, thus placing demands on the way in which site suitability under a changing climate is assessed ([44]).
Site suitability is evaluated for forest tree species by population mapping, growth demands and productivity typification. Norway spruce forms two distinctive populations, the Northern European and Central European-Balkan populations ([10]). The Central European population is further divided into individual mountain ranges due to the varying impacts of Quaternary climate changes. Warm Quaternary interglacial periods pushed spruce populations into the mountain spruce vegetation tier or into the boreal zone after the retreat of the continental glaciation. In contrast, spruce survived ice ages in deep intermountain valleys ([42]). Global warming has mostly affected submountain forests, while the stock increment of mountain forests has increased ([16]). Similarly, the spruce submountain population appears to be more vulnerable to warming than broadleaved tree species.
The Central European-Balkan submountain spruce population was originally composed of remnants after the Quaternary cold periods ([34]). The significance of the residual populations lies in the fact that the spread of spruce in the interglacial times commenced with these populations. Spruce may easily reproduce by the transfer of pollen and seeds by the wind. Seeds are quickly taken up in both the open areas and the shade of older forest stands. A characteristic difference between spruce and other Central European coniferous trees is a shallow root system, which makes spruce demanding in regard to soil moisture; this regulates the transpiration flow, such as in the humid environment, to avoid suffering from stagnant soil unoxygenated water ([7]).
Submountain spruce populations survive in cold valleys thanks to constant soil moisture and the reduced competitiveness of broadleaved trees ([18]). Under such conditions, the occurrence of spruce contributes to forest adaptability by maintaining biodiversity. Spruce complements the tree composition and co-determines niches for soil organisms by increasing the supply of more slowly decomposing humus ([44]). Soil organic accumulation around weathered rock particles increases the site water-holding capacity ([21]). Increasing the soil water-holding capacity reduces the runoff rate, thus protecting the site from desiccation and simultaneously mitigating the effects of extraordinary rainfall.
The direct tree effect on soil properties is usually limited to surface humus, and it declines rapidly with increasing the depth through mineral horizons. Spruce is less demanding in regard to subsoil acidity, despite it accelerates local soil acidification ([28]). While conifer humus acidifies soil due to reduced litter decomposition under higher C/N and acid-forming cation activities, nutrient access through rhizosphere bacterial activity is independent of tree species ([6], [43]). In alkaline subsoil, spruce has proven to be less competitive than broadleaved trees, which accelerate seed germination through more efficient decomposition of leaf litter in the presence of Ca2+ ([44]).
Despite its tolerance to acidic soils, Norway spruce is very sensitive to acidic atmospheric deposition. Environmental loads due to acidification and desiccation owing to global warming have caused both extensive spruce stand decline and weakened resistance against pathogenic fungi and harmful insects ([19]). Declining submountain spruce populations affect both managed stands and indigenous communities. The decline of residual spruce populations has subsequently threatened the ability of submountain forests to adapt to global climate change ([20]).
The aim of the present study was to distinguish between suitable and unsuitable spruce sites in the Central European submountain altitudes through different correlations among soil properties. Central European forests have been extensively transformed into spruce plantations, which have subsequently been affected by the simultaneous effects of acid deposition and climate change ([25]). Soil properties, however, have remained part of the permanent growth conditions, which do not rapidly alter as a consequence of the aftermath transformation of tree species composition. Correlations between soil water-holding capacity and soil chemistry indicate the limits of forest tree species competitiveness ([5]). Thus, soil correlations represent ecosystem property substitutions, where the lack of one quantity allows tree species occurrence due to the excess of another quantity along the margins of suitable sites ([17]). Soil property substitutions cause differences between forest communities with the same dominant tree species on the one hand and between sites with different tree species on the other, despite the same soil group and relief ([39]).
Tree species survival at a site is supported through biochemically controlled access to limited nutrients. Biochemical nutrient access supports tree species through the formation of extracellular enzyme complexes with soil particles ([40]). The effectiveness of enzyme complexes is related to the time that they remain active outside the mother cells. The period of extracellular activity depends on the soil organic matter content and on the rock at the site. The enzyme complexes increase the dependence of nutrient availability on stable growth conditions. Thus, plant effects on soil properties are less significant due to enzyme complex activity ([4]). In this way, comparing the effects of soil particles on enzyme activity can help to indicate suitable growth conditions for spruce and broadleaved stands ([29]).
Material and methods
Submountain forests
Several submountain sites of Norway spruce were selected throughout the territory of the Czech Republic (CR), where extensive spruce stand cultivations can be found. The natural proportion of Norway spruce in the CR was 11.2%. The present-day proportion of spruce is 49.5% due to plantations outside of its natural sites ([30]). Natural spruce populations are generally divided into submountain (< 700 m a.s.l), mountain (700-1100 m a.s.l.) and high mountain (> 1100 m a.s.l.) populations ([13]). The spruce proportion was only 0.19% in the submountain forests, 20% in the mountain forests and over 79% in the high-mountain forests. The current proportion of spruce is almost 27% in submountain forests, 68% in mountain forests and 88% in high-mountain forests ([22]). At the same time, almost 70% of Central European submountain forests were naturally formed by common beech (Fagus sylvatica) and oaks (Quercus sp.), while their current representation in submountain altitudes is only 10% ([12]).
Soil survey
Field sampling
The suitable spruce sites were distinguished by comparing selected pairs of the involved mature mixed stands with natural spruce regeneration and pure broadleaved and spruce stands larger than 0.25 ha located in the same soil series at altitudes < 700 m a.s.l. during 2017-2020. The stands were selected from forest areas with natural submountain spruce populations ([18]). The natural submountain spruce population in the territory of the CR consists of 11 subpopulations, from which we selected eight with protected spruce stands ([9] - Fig. 1). Each of the eight subpopulations was surveyed using repeated selection of representative protected stand types in an 8 × 8 km grid ([20]). Spruce and mixed stands were selected from gene platforms in database ERMA 2 managed by the Forest Management Institute Brandýs nad Labem, while broadleaved stands were selected in spatially related reserves. Each forest stand pair was acquired by the process of repetition. The longest feasible distance between each forest stand type pair in the grid was based on criteria at the national level for temperate forest monitoring. The selection of representative forest stand pairs was based on inventory survey criteria ([11]). The application of pairs allowed for a more accurate calculation of average values than medians in small samples ([24]).
Fig. 1 - Localities of forest stand survey related to Norway spruce (Picea abies) distribution in the Czech Republic. For further detail information on the survey (sub)populations, see Tab. 1.
The soil under spruce natural regeneration was sampled where spruce formed advanced growth (> 20 cm tall) to the understory (< 10 m tall), including > 25% of the total stand canopy. In each case, common soil characteristics were assessed using the same types of subsoil and relief. The soil series were characterized by representation of matrices and patches of soil groups according to WRB-ISSS-ISRIC ([41]). Relief was typed using classification of altitude intervals and relative elevation ([33]). Spruce stands were selected from homogeneous forests. Broadleaved stand type was chosen from forests where beech or oak represented > 50% of the total stand basal area ([23]).
Soil analysis
The soil survey included sampling and chemical analysis of the surface humus (H-horizon) and A-horizon in the mixed samples from three pits located on a diagonal line inside the plot at each selected forest stand. Plots were defined by a perimeter half the mean stem height from the stand margin ([36]). Each soil sample was measured for pH, cation exchange capacity (CEC) and base saturation (BS) according to EMEP-LRTAP procedures ([32]), for organic carbon (Corg), total nitrogen (Ntot) and microbial carbon (Cmic) contents spectrophotometrically ([45]) and for activities of urease, phosphomonoesterase and catalase. The urease ([26]) and acid phosphomonoesterase activities ([37]) were determined by comparative incubation, while the catalase activity was determined volumetrically ([3]). Ureases and phosphatases access shortcoming nitrogen and phosphorus substances under aerobic conditions in the presence of catalase ([48]). The A-horizons were also characterized gravimetrically to determine particle-size composition according to the USDA ([8]), bulk (Dd) and specific (Ds) densities, water-holding capacity (WHC), porosity (P) and aeration (A) ([39]).
Statistical assessment
Site suitability for submountain spruce was assessed using linear discriminant analysis (LDA) among the stand types and forest areas selected. Assumptions for using LDA were validated through tests on input data normality and through analysis of variance between covariance matrices from soil properties in comparable stand types and forest areas at p < 0.05. Data normality was verified by assessing of skewness (A1) and elevation (E1). A tolerable level of departure from normal data distribution was confirmed by homogenous variance between covariance matrices ([15]). The properties in H- or A-horizons suitable for spruce were estimated by discriminant functions in the following form ([38] - eqn. 1):
where i is the number of forest stands sampled; q is the number of soil properties; Zi is the standardized classification coefficient of the forest stand type; a is the discriminant vector parameter determined by the ratio of differences in the independent variables compared (xj - xj+1) with the covariance matrix (Q) and x is the standardized independent variable (eqn. 2):
where j is the number of classified stand types. The threshold point for the correspondence between soil properties and stand type (C) was determined by means of the semisum between averages of classification coefficients from the forest stands with the value of stand type discrimination (Zj - eqn. 3):
where (eqn. 4):
The effects of H-horizons were assessed using chemical properties, but the effects of A-horizons were appraised from a series including soil properties, altitude and relief slope. While H-horizon properties depend on the chemical composition of plant litter, A-horizon properties are formed by the combined effect of organic matter and subsoil weathering which can be different along relief exposure ([11], [29], [4]). The function parameters among forest areas were used to estimate the subsoil effect on spruce site suitability.
The parameter reliability at discriminant functions was assessed using separability from the proportion of correctly classifiable stands ([47]).
Results
Representative forest populations were found on four rock types, five relief types and eight types of soil associations (Tab. 1). Three populations in the Western Sudetes, Sázava and Javorice Highlands all occurred on acid plutonites. Similarly, four stand populations occurred on fertile outermost sedimentary rocks. The Krivoklát and karst populations occurred primarily on limestones, while the North Bohemian and Beskidian (West Carpathian) stand pairs were defined as on sandstones. However, the occurrence of stand populations in individual relief types did not follow the division of rock types. The West Sudeten and Sázava populations were both located within indented plateaus, while the Javorice and Beskidian populations were both identified on steep slopes. Between these populations were the Krivoklát and Below-Beskidian populations, both defined as flat highlands (Tab. 1).
Tab. 1 - Altitudinal ranges (± standard deviation) among forest stand type subpopulations compared to bedrock, relief and main soil series.
Pair | Population | Bedrock | Relief | Soil series | Altitude (m a.s.l.) |
Broadleaved (m a.s.l.) |
Mixed (m a.s.l.) |
Spruce (m a.s.l.) |
---|---|---|---|---|---|---|---|---|
1 | North-Bohemian | Sandstone | Rock city | Arenic Podzols | 312 ± 63 | 324 ± 65 | 310 ± 66 | 302 ± 75 |
2 | West-Sudeten | Acid plutonite | Broken plateau | Skeletic Podzols | 488 ± 26 | 501 ± 31 | 486 ± 24 | 478 ± 23 |
3 | Krivoklát | Limestone | Highland | Endoeutric Cambisols | 463 ± 72 | 482 ± 83 | 456 ± 84 | 453 ± 66 |
4 | Sázava | Acid plutonite | Broken plateau | Haplic Cambisols | 460 ± 50 | 476 ± 49 | 468 ± 54 | 437 ± 52 |
5 | Javorice | Acid plutonite | Slope | Dystric Cambisols | 583 ± 15 | 586 ± 5 | 583 ± 11 | 580 ± 25 |
6 | Karstic | Limestone | Valley | Rhodi-rendzic Leptosols | 424 ± 57 | 447 ± 71 | 396 ± 44 | 429 ± 56 |
7 | Below-Beskidian | Marl flysch | Highland | Stagnic Retisols | 353 ± 76 | 357 ± 78 | 361 ± 93 | 341 ± 77 |
8 | Beskidian | Sandstone | Slope | Skeletic Cambisols | 647 ± 31 | 676 ± 26 | 640 ± 29 | 626 ± 38 |
Typically, the North Bohemian stands were lowest-lying, while the Beskidian stands were located at the highest altitudes. The broadleaved stands were usually located at higher altitudes, while the spruce stands were at lower altitudes, except for the Below-Beskidian population. Mixed stands were defined as below average in the North Bohemian, West Sudeten, Krivoklát, Javorice, karst and Beskidian populations. The mixed stands in the Sázava and Below-Beskidian areas were highly above average. The highest-lying mixed stand was located in the Below-Beskidian area, while the lowest-lying mixed stand was in the Moravian Karst. At the same time, the broadleaved and mixed stands tended to have a higher relief slope than spruce stands, although the highest slope occurred in the mixed stands.
The representative forest stands covered twenty-seven soil groups in thirteen series. Nevertheless, only the Cambisols (59.4%) and Leptosols (16.7%) included more than 75% of the occurring soil groups. Similar to Leptosols, Podzols were found under 13.5% of forest stands, while Luvisols (4.2%) and Retisols (5.2%) together did not cover even 10% of the forest stands. Global soil series indicated Podzols as the predominant occurrence in the North Bohemian and West Sudeten populations, while Cambisols were divided into the Krivoklát/Sázava/Javorice populations and the Beskidian population. This division was mainly caused by the occurrences of Retisols and Leptosols on the border between the Bohemian Massif and the Outer Carpathian Depressions.
The soil properties formed an antagonistic value series from the broadleaved to spruce stands. While ascending values of soil properties clearly differed between H- and A-horizons in broadleaved and spruce stands, the descending values were relatively similar. Physico-chemical properties and enzymatic activities showed the highest departure from normal distribution of data. In contrast, altitude, coarse-particle contents in topsoil horizons and organic matter at surface humus preserved data normality (Tab. 2). Broadleaved stands were characterized by the highest levels of soil acidity, BS, Cmic and enzyme activities in surface humus. A-horizons under the broadleaved stands were typical by localization at the highest altitudes, the greatest Ds, pH and acid phosphomonoesterase (APMEA) and catalase (CA) activities. In contrast, spruce humus was characterized by high contents of carbon, nitrogen and cation exchange capacity. Similarly, the A-horizons under spruce stands were characterized by the greatest contents of sand, clay, Dd, CEC and WHC.
Tab. 2 - Soil properties (± standard deviation) at forest stand type populations. CEC: cation exchange capacity (cmol+ kg-1); BS: base saturation (%); Corg: organic carbon (%); Cmic: microbial carbon (%); Ntot: total nitrogen (%);UA : urease activity (mg hour-1); APMEA: acid phosphomonoesterase activity (mg hour-1); CA: catalase activity (mg hour-1); Altitude (m a.s.l.); Slope (°); Sand, Silt and Clay (%); Dd: bulk density (g cm-3); Ds: specific density (g cm-3); WHC: water-holding capacity (%); Porosity (%); Aeration (%); A1: skewness test criterion; E1: elevation test criterion. (*) significant departure from normality (p < 0.05).
Horizon | Quality | Broadleaved | Mixed | Spruce | Mean | A1 | E1 |
---|---|---|---|---|---|---|---|
H | pH | 4.72 ± 0.94 | 4.51 ± 1.18 | 4.25 ± 1.12 | 4.50 ± 1.09 | 5.10* | 0.72 |
CEC | 29.75 ± 35.48 | 28.26 ± 19.23 | 32.99 ± 42.29 | 30.34 ± 33.45 | 18.06* | 45.35* | |
BS | 67.02 ± 27.48 | 58.15 ± 31.18 | 50.98 ± 28.56 | 58.72 ± 29.55 | 0.21 | 2.96* | |
Corg | 20.97 ± 5.19 | 21.32 ± 4.48 | 22.60 ± 4.89 | 21.63 ± 4.86 | 1.61 | 2.40* | |
Cmic | 1.06 ± 1.04 | 0.76 ± 0.62 | 0.68 ± 0.59 | 0.83 ± 0.79 | 5.02* | 3.38* | |
Ntot | 1.34 ± 0.35 | 1.33 ± 0.32 | 1.37 ± 0.38 | 1.35 ± 0.35 | 0.17 | 1.45 | |
UA | 109.81 ± 114.49 | 92.72 ± 100.34 | 64.92 ± 79.53 | 89.15 ± 99.86 | 4.92* | 0.49 | |
APMEA | 418.57 ± 348.05 | 352.39 ± 252.99 | 317.51 ± 248.57 | 362.82 ± 286.97 | 6.29* | 5.15* | |
CA | 108.51 ± 171.08 | 84.56 ± 132.78 | 99.03 ± 150.33 | 97.37 ± 150.92 | 9.93* | 12.49* | |
A | Altitude | 492.28 ± 140.72 | 474.94 ± 138.46 | 455.34 ± 114.07 | 474.19 ± 131.13 | 1.71 | 0.03 |
Slope | 13.15 ± 8.96 | 13.83 ± 10.08 | 12.24 ± 10.65 | 13.07 ± 9.84 | 4.76* | 1.06 | |
Sand | 34.67 ± 13.29 | 31.75 ± 17.47 | 39.68 ± 21.55 | 35.37 ± 17.88 | 0.48 | 0.40 | |
Silt | 34.74 ± 9.36 | 36.90 ± 10.60 | 30.73 ± 13.21 | 34.12 ± 11.35 | 0.06 | 0.51 | |
Clay | 3.74 ± 3.08 | 4.83 ± 5.18 | 5.24 ± 6.22 | 4.60 ± 4.99 | 7.30* | 6.11* | |
Dd | 0.86 ± 0.22 | 0.79 ± 0.25 | 0.91 ± 0.23 | 0.86 ± 0.24 | 0.59 | 0.01 | |
Ds | 2.26 ± 0.17 | 2.25 ± 0.22 | 2.23 ± 0.24 | 2.25 ± 0.21 | 8.83* | 13.02* | |
WHC | 34.47 ± 6.27 | 33.75 ± 10.55 | 31.79 ± 8.10 | 33.33 ± 8.45 | 1.34 | 1.30 | |
Porosity | 62.77 ± 8.04 | 65.81 ± 9.00 | 58.94 ± 10.14 | 62.47 ± 9.44 | 1.47 | 2.24* | |
Aeration | 53.19 ± 17.17 | 56.67 ± 15.66 | 49.10 ± 17.24 | 52.95 ± 16.82 | 2.65* | 0.58 | |
pH | 4.34 ± 1.02 | 4.30 ± 1.03 | 4.20 ± 1.07 | 4.28 ± 1.03 | 8.32* | 6.43* | |
CEC | 16.66 ± 14.23 | 16.22 ± 11.97 | 23.89 ± 44.41 | 18.92 ± 27.73 | 24.65* | 88.93* | |
BS | 36.97 ± 27.79 | 40.33 ± 31.00 | 34.22 ± 26.87 | 37.17 ± 28.42 | 5.75* | 0.96 | |
Corg | 9.27 ± 4.47 | 8.33 ± 3.99 | 8.93 ± 4.24 | 8.84 ± 4.21 | 3.41* | 0.52 | |
Cmic | 0.26 ± 0.26 | 0.21 ± 0.21 | 0.21 ± 0.31 | 0.23 ± 0.26 | 7.79* | 7.85* | |
Ntot | 0.53 ± 0.28 | 0.47 ± 0.25 | 0.49 ± 0.39 | 0.50 ± 0.31 | 8.46* | 12.27* | |
UA | 52.33 ± 72.30 | 40.06 ± 51.65 | 31.13 ± 62.22 | 41.17 ± 62.58 | 12.87* | 22.09* | |
APMEA | 164.33 ± 97.79 | 153.15 ± 111.88 | 135.57 ± 106.68 | 151.02 ± 105.17 | 7.82* | 26.88* | |
CA | 42.76 ± 66.85 | 38.88 ± 57.86 | 32.58 ± 46.15 | 38.07 ± 57.13 | 11.57* | 63.51* |
The lowest values of humus CEC, Ntot and CA in mixed stands disrupted the unilateral arrangement of soil property values in proportion to the representation of broadleaved trees. The A-horizons under mixed stands were characterized by the lowest contents of sand, Dd, CEC, carbon, nitrogen and urease activity (UA). In contrast, the mixed stands were located on the steepest slopes with the highest silt contents and greatest porosity, aeration and base saturation. Broadleaved stands were characterized by high Ds and WHC and by high contents of carbon, nitrogen and enzyme activities in A-horizons.
Soil horizon properties tended to differ more due to subsoil than tree species composition. The soil properties of A-horizons indicated the suitability of spruce sites much more reliably than humus properties. Individual forest populations were also more dissimilar to each other than pure spruce or broadleaved types. The broadleaved and mixed forest stands differed from each other more than from spruce stands, while soil properties in mixed stands appeared to be the most distinct (Tab. 3). Simultaneously, the distinctness of mixed stands was similar in both organic and A-horizons. All forest populations had soil properties distributed along acidic sites above 500 m a.s.l. and nutrient-rich sites below 500 m a.s.l. (Fig. 2). Variances of covariances confirmed that departure from data normality of soil properties did not reduce discriminant function reliability. The covariance homogeneity corresponded with increasing subsoil effects on soil property distributions (Tab. 4).
Tab. 3 - Separability between soil properties in the humus (H-) and A-horizons of submountain spruce population and forest stand types.
Discriminant | Variable | H | A |
---|---|---|---|
Population | North-Bohemian | 66.67 | 100.00 |
West-Sudeten | 58.33 | 100.00 | |
Krivoklát | 50.00 | 75.00 | |
Sázava | 58.33 | 72.73 | |
Javorice | 75.00 | 91.67 | |
Karstic | 58.33 | 75.00 | |
Below-Beskidian | 41.67 | 66.67 | |
Beskidian | 50.00 | 100.00 | |
Total | 57.29 | 85.26 | |
Stand type | Broadleaved | 46.88 | 56.25 |
Mixed | 62.50 | 62.50 | |
Spruce | 34.38 | 51.61 | |
Total | 47.92 | 56.84 |
Tab. 4 - Means ± standard deviations in covariance matrices for soil properties in the humus (H-) and A-horizons of submountain spruce populations and forest stand types. For further detail information about covariance, see Tab. S2 and Tab. S4 in Supplementary material.
Discriminant | Variable | H | A |
---|---|---|---|
Population | North-Bohemian | -14.469 ± 63.248 | 970 ± 18.117 |
West-Sudeten | 34.213 ± 15.2452 | -363 ± 8.918 | |
Krivoklát | 34.024 ± 159.074 | 406 ± 11.181 | |
Sázava | -45.314 ± 192.705 | -336 ± 4.101 | |
Javorice | -1.469 ± 20.198 | 690 ± 7.559 | |
Karstic | -34.939 ± 287.003 | -446 ± 19.380 | |
Below-Beskidian | -30.290 ± 130.634 | -436 ± 5.872 | |
Beskidian | -10.150 ± 131.809 | 32 ± 4.626 | |
Stand type | Broadleaved | -46.306 ± 186.331 | -549 ± 6.435 |
Mixed | -13.722 ± 71.891 | -578 ± 10.680 | |
Spruce | -21.300 ± 117.299 | 859 ± 5.785 |
The subsoil divided parameter directions at discriminant functions between stand types. The broadleaved stands were characterized by the highest number of positively directional parameters. Altitude, hydrophysical bulk and specific densities, water-holding capacity, porosity and aeration, pH, Cmic, Ntot and enzyme activities covered 59% of the properties evaluated. The mixed stands displayed positive directionality in 53% of soil properties, and 47% displayed negative directionality. The spruce stands were characterized by positive directionality in just 18% of soil properties, while 82% were negative. The parameters of slope, granularity, Dd, WHC, porosity, aeration, base saturation, Ntot and APMEA directly specified sites of the mixed stands, while spruce sites were specified using only the positive directionality of particle size, cation exchange capacity and Corg. Mixed stands were characterized by the highest proportion of equally directional soil property parameters. Only slope and BS were similarly directional between spruce and broadleaved stands. Altitude, particle size, Ds, pH, Cmic and urease and catalase activities were equally directional between mixed and spruce stands, while Dd, WHC, porosity, aeration, CEC, Corg, Ntot and APMEA were equally directional between mixed and broadleaved stands (Tab. 5).
Tab. 5 - Function parameters among forest stand type populations in relation to discriminative semisums. For the definitions of variables, see Tab. 2.
Function | Variable | Broadleaved | Mixed | Spruce |
---|---|---|---|---|
Parameter | Altitude | 0.32 | -0.02 | -0.32 |
Slope | -0.09 | 0.37 | -0.19 | |
Sand | -1.20 | 0.53 | 0.77 | |
Silt | -0.59 | 0.56 | 0.08 | |
Clay | -1.11 | 0.09 | 1.09 | |
Dd | 0.08 | 0.53 | -0.54 | |
Ds | 0.48 | -0.09 | -0.39 | |
WHC | 0.09 | 0.06 | -0.16 | |
Porosity | 0.14 | 0.88 | -0.94 | |
Aeration | 0.02 | 0.20 | -0.24 | |
pH | 0.51 | -0.38 | -0.13 | |
CEC | -0.67 | -0.34 | 0.97 | |
BS | -0.47 | 0.64 | -0.11 | |
Corg | -0.01 | -0.37 | 0.40 | |
Cmic | 0.26 | -0.23 | -0.03 | |
Ntot | 0.68 | 0.40 | -1.06 | |
UA | 0.59 | -0.03 | -0.63 | |
APMEA | 0.03 | 0.02 | -0.05 | |
CA | 0.21 | -0.15 | -0.09 | |
Interception | -1.40 | -1.33 | -1.61 | |
Stand | Broadleaved | -0.77 | -0.84 | -0.66 |
Mixed | -0.84 | -0.91 | -0.74 | |
Spruce | -0.66 | -0.91 | -0.56 |
Discussion
The properties of soil A-horizons in the Central European submountain localities distinguished sites suitable for Norway spruce (P. abies) cultivation outside of the species vegetation tier; this method is a tool for assessing adaptations in forest tree species composition to environmental change.
Sustainable forest adaptation to environmental change is generally based on close-to-natural management of mixed stands. The close-to-nature cultivation of mixed forest stands protects both biodiversity and wood production stability ([14]). However, representative mixed forest stands were found to affect soil properties less than the locality of each tree population. Locality significantly affected soil properties more through altitude than through geographical distance. The sites of the submountain spruce populations varied along a gradient of nutrient-rich soils at < 500 m a.s.l. to acidic soils between 500-700 m a.s.l. Nevertheless, soil properties in mixed forest stands were more similar to those in spruce stands than to those in broadleaved stands, which corresponds with assumptions of a wider presence of admixed spruce at submountain altitudes ([27], [13], [44]). Soil property differentials between mixed and broadleaved stands, being inside the same soil series or forest area or under the same dominant broadleaved species, are likely to be the consequence of past disturbances in the ecosystem structure ([28], [46], [32]).
Discriminant analysis divided the forest stands into three clusters comprising Beskidian populations, North Bohemian-Below-Beskidian and Czech-Moravian populations, including karst and highland populations. Beskidian stands were located on sandstones at > 607 m a.s.l., while North Bohemian-Below-Beskidian stands on sandstones to marl flysch at 222-469 m a.s.l. The Czech-Moravian cluster formed the boundary between two extremely occurring forest populations in the altitudinal range of 344-601 m a.s.l. on contrasting plutonites and limestones. The A-horizons in mixed forest stands were the least sandy with low Dd, cation exchange capacity, Corg, and Ntot and the lowest urease activity. On the other hand, they tended to be steepest and had the highest silt contents, porosity, aeration and base saturation. Mixed and spruce stands were characterized by similar soil particle size, Ds, pH, Cmic and urease as well as catalase activity. Although soil pH, Cmic, UA and CA are influenced by the transformation of tree species composition, the correlations with particle size and Ds suggests the dominant effect of the parent substrate ([46]). In such cases, organic substances are stabilized by mineral particles from the parent substrate, allowing maintenance of biochemical activity despite disruption of the original forest structure ([4]).
Soil particle size, specific density, pH, Cmic, UA and CA defined optimal soil conditions for the occurrence of submountain Norway spruce. The margins of submountain spruce sites were represented by average WHC > 34%, above-average APMEA > 151 μg h-1, soil porosity > 63% and aeration > 53%, but below-average Dd < 0.86 g cm-3, CEC < 18 cmol+ kg-1, Corg< 9% and Ntot< 0.5%. The indicative A-horizon properties suggested that optimization of forest tree species composition is, on the one hand, inevitably related to the protection of soil functions and, on the other hand, is also related to protection of local tree species population. The urease and phosphatase activities involved in the decomposition of nitrogenous and phosphorus compounds are particularly indicative of unique natural complexes of plant growth conditions ([2]). The soil environment provides robust bioindicators of forest growth, transformation and filtration ecosystem functions. At the same time, landscape transformations and climate change threaten soil functions to the point of ecosystem collapse ([31]). Fundamental pro-growth soil functions are triggered by symbiotic and commensal microorganisms. Soil microorganisms enzymatically decompose organic matter into nutrients that they provide to plants ([40]). Nonetheless, the soil group and land use affect the abilities of plants and microorganisms to stabilize soil organic matter.
Access to phosphorus is mostly conditioned by acceptable forms bound at soil organic matter ([37]). In addition to the total organic matter content, the phosphorus release from dead organic matter depends on soil moisture and, less so, on the content of aluminum oxides. Aluminum compounds reduce microbial activity and subsequent mineralization efficiency and thus support soil organic matter stabilization ([1]). In Chernozems and Luvisols, suitable conditions for microbial activity are reflected in the high proportion of microbial components in stabilized soil organic matter, while less suitable conditions in Cambisols or Podzols are characterized by a higher proportion of plant litter ([4]). In our study, the soil environmental effects on enzymatic activity were also evident through clustering of forest stand populations between more suitable nutrient-rich sites and less suitable acidic sites.
Our comparison of spruce populations in Czech Republic has shown that their ability to protect soil functioning is associated with both naturalness and preservation of ecosystem diversity. The transformation to managed forests has significantly affected the spruce gene pool ([18]). Norway spruce was planted outside its natural sites, particularly far from the Central European population. Therefore, preserved local natural spruce stands are essential for the restoration of forest natural gene pools to support adaptation to climate change ([25]). However, spruce genetic diversity in the territory of the Czech Republic is not completely reflected by the distribution of submountain sites. Mountain and submountain spruce populations are not genetically different ([13]). Nevertheless, natural spruce populations tend to be more genetically diverse than planted stands. The magnitude of natural genetic differences roughly corresponds to the geographical distance between native spruce populations ([9]). The geographical dependence between different spruce genetic diversity and suitable sites was maintained, particularly in the instance of Bohemian occurrences. In contrast, this geographical dependence was disregarded alongside borders of the CR between the nearby North Bohemian and West Sudeten populations and between the Below-Beskidian and Beskidian populations. Despite this, the indication of forest naturalness through site characteristics seems to be applicable both for threat assessment and for defining sustainable planting of Norway spruce.
Conclusion
The correlations of the soil particle size and specific density with biochemical properties indicated suitable conditions for mixed occurrence of Norway spruce (P. abies) in the Central European submountain forests. The limits of the submountain spruce population were located along above-average soil acid phosphomonoesterase activity, porosity and aeration at below-average soil bulk density, cation exchange capacity and Corg and Ntot contents. The suitable submountain spruce sites were divided between nutrient-rich soils at < 500 m a.s.l. and acidic soils at altitudes of 500-700 m a.s.l. in clusters comprising the Bohemian highlands, North Bohemian rock cities and the Outer Western Carpathians. The applied discriminant analysis of soil hydrophysics, physical chemistry and biochemistry was proven to be a useful tool for defining naturalness as well as forest threats.
Acknowledgements
The presented study has been supported by the project LDF_TP_2021006 of the Internal Grant Agency of Mendel University in Brno, Czech Republic.
References
Gscholar
Gscholar
Gscholar
Gscholar
Gscholar
Gscholar
Gscholar
Gscholar
CrossRef | Gscholar
Authors’ Info
Authors’ Affiliation
Jirí Volánek 0000-0003-3629-4971
Ladislav Holík 0000-0002-2357-9487
Marie Balková 0000-0003-4796-4491
Valerie Vranová 0000-0003-0931-2345
Department of Geology and Soil Science, Faculty of Forestry and Wood Technology, Mendel University, Zemedelská 3, CZ-613 00 Brno (Czech Republic)
Department of Forest Botany, Dendrology and Geobiocoenology, Faculty of Forestry and Wood Technology, Mendel University, Zemedelská 3, CZ-613 00 Brno (Czech Republic)
Corresponding author
Paper Info
Citation
Samec P, Volánek J, Holík L, Rychtecká P, Balková M, Vranová V (2023). The effect of soil conditions on submountain site suitability for Norway spruce (Picea abies Karst.) in Central Europe. iForest 16: 210-217. - doi: 10.3832/ifor4262-016
Academic Editor
Luigi Saulino
Paper history
Received: Nov 10, 2022
Accepted: May 22, 2023
First online: Jul 31, 2023
Publication Date: Aug 31, 2023
Publication Time: 2.33 months
Copyright Information
© SISEF - The Italian Society of Silviculture and Forest Ecology 2023
Open Access
This article is distributed under the terms of the Creative Commons Attribution-Non Commercial 4.0 International (https://creativecommons.org/licenses/by-nc/4.0/), which permits unrestricted use, distribution, and reproduction in any medium, provided you give appropriate credit to the original author(s) and the source, provide a link to the Creative Commons license, and indicate if changes were made.
Web Metrics
Breakdown by View Type
Article Usage
Total Article Views: 7991
(from publication date up to now)
Breakdown by View Type
HTML Page Views: 6923
Abstract Page Views: 504
PDF Downloads: 498
Citation/Reference Downloads: 0
XML Downloads: 66
Web Metrics
Days since publication: 272
Overall contacts: 7991
Avg. contacts per week: 205.65
Article Citations
Article citations are based on data periodically collected from the Clarivate Web of Science web site
(last update: Feb 2023)
(No citations were found up to date. Please come back later)
Publication Metrics
by Dimensions ©
Articles citing this article
List of the papers citing this article based on CrossRef Cited-by.
Related Contents
iForest Similar Articles
Research Articles
Effects of arbuscular mycorrhizal fungi on microbial activity and nutrient release are sensitive to acid deposition during litter decomposition in a subtropical Cinnamomum camphora forest
vol. 16, pp. 314-324 (online: 13 November 2023)
Research Articles
Impact of deforestation on the soil physical and chemical attributes, and humic fraction of organic matter in dry environments in Brazil
vol. 15, pp. 465-475 (online: 18 November 2022)
Research Articles
Soil stoichiometry modulates effects of shrub encroachment on soil carbon concentration and stock in a subalpine grassland
vol. 13, pp. 65-72 (online: 07 February 2020)
Research Articles
Dynamics of soil organic carbon (SOC) content in stands of Norway spruce (Picea abies) in central Europe
vol. 11, pp. 734-742 (online: 06 November 2018)
Research Articles
Potential relationships of selected abiotic variables, chemical elements and stand characteristics with soil organic carbon in spruce and beech stands
vol. 14, pp. 320-328 (online: 09 July 2021)
Research Articles
Arbuscular mycorrhizal fungal symbiosis with Sorbus torminalis does not vary with soil nutrients and enzyme activities across different sites
vol. 8, pp. 308-313 (online: 03 September 2014)
Research Articles
Impacts of Norway spruce (Picea abies L., H. Karst.) stands on soil in continental Croatia
vol. 12, pp. 511-517 (online: 02 December 2019)
Research Articles
Effect of different dolomitic limestone dosages on soil respiration in a mid-altitudinal Norway spruce stand
vol. 12, pp. 357-365 (online: 05 July 2019)
Research Articles
Soil CO2 efflux in uneven-aged and even-aged Norway spruce stands in southern Finland
vol. 11, pp. 705-712 (online: 06 November 2018)
Research Articles
Soil C:N stoichiometry controls carbon sink partitioning between above-ground tree biomass and soil organic matter in high fertility forests
vol. 8, pp. 195-206 (online: 26 August 2014)
iForest Database Search
Search By Author
Search By Keyword
Google Scholar Search
Citing Articles
Search By Author
Search By Keywords
PubMed Search
Search By Author
Search By Keyword